Theoretical and experimental analysis of a boost converter
- Post by: SOAPHYS-KZ
- 17 juin 2021
- Comments off
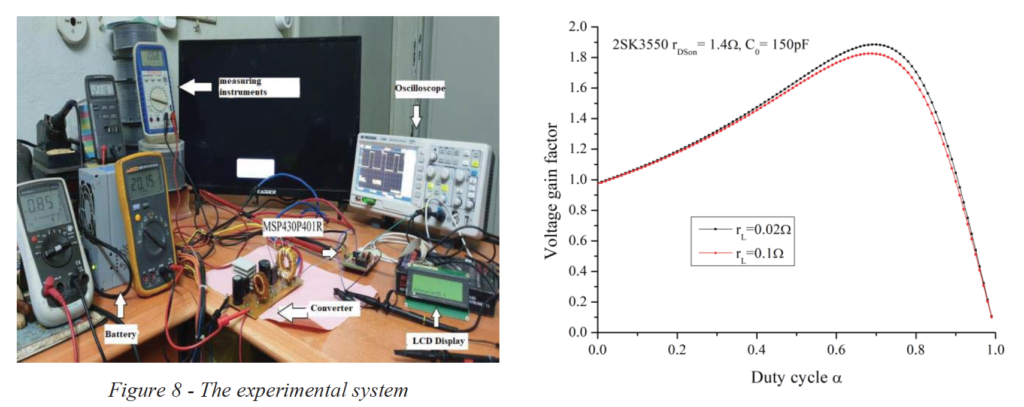

http://dx.doi.org/10.46411/jpsoaphys.2020.02.28
Section de la parution: Informations de publication
J. P. Soaphys, Vol 2, N°2 (2020) C20A28; 25 Juin 2021
Pages : C20A28-1 à C20A28-9
Information sur les auteurs
Badiane Modou 1*
Honadia Prince Abdoul Aziz 2,
Barro Fabé Idrissa 3
1 Semiconductors and Solar Energy Laboratory, Department of Physics to the Faculty of Science and Technique, Cheikh Anta Diop University, Dakar, Senegal,
2 Thermal and Renewable Energies Laboratory, Department of Physics to Polytechnic University Center of kaya, Burkina Faso,
3 Semiconductors and Solar Energy Laboratory, Department of Physics to the Faculty of Science and Technique, Cheikh Anta Diop University, Dakar, Senegal,
*To whom correspondances should be addressed. E-mail: *modoubadiane99@gmail.com
Une étude théorique et expérimentale d’un convertisseur boost conventionnel est présentée. Sur la base du comportement réel des composants, le modèle de convertisseur élévateur traitant aussi bien des pertes inductives et capacitives que des pertes de commutation est introduit. À partir de ce modèle, les expressions analytiques détaillées du facteur de gain en tension et du rendement de conversion sont établies en tenant compte des pertes dues aux résistances parasites et aux pertes de commutation. Le comportement du convertisseur est ensuite analysé par simulation du facteur de gain en tension et du rendement de conversion en fonction du rapport cyclique. Le prototype du convertisseur a été mis en oeuvre et un ensemble de mesures expérimentales a été effectué ; ces mesures ont permis de démontrer que les modèles théoriques proposés étaient fiables pour une large gamme de rapport cyclique du convertisseur boost.
Abusorrah, A., Al-Hindawi, M. M., Al-Turki, Y., Mandal, K., Giaouris, D., Banerjee, S., Voutetakis, S. and Papadopoulou, S. (2013) Stability of a boost converter fed from photovoltaic source, Solar Energy, 98, 458-
471. https://doi.org/10.1016/j.solener.2013.09.001
Ayop, R. and Tan, C. W. (2018) Design of boost converter based on maximum power point resistance for photovoltaic applications, Solar Energy, 160, 322–335. https://doi.org/10.1016/j.solener.2017.12.016
Branko L. Dokić, Branko Blanuša, (2015) Power Electronics: Converters and Regulators, 3rd Edition, Springer International Publishing.
Das, M., Agarwal, V. (2012) A novel, high efficiency, high gain, front end dc-dc converter for low input voltage solar photovoltaic applications, Proceedings of the 38th Annual Conference of the IEEE IECON, 5744– 5749.
Dash, S. S. and Nayak, B. (2015) Control analysis and experimental verification of a practical dc–dc boost
converter, Journal of Electrical Systems and Information Technology, 2, 378-390. https://doi.org/10.1016/j.jesit.2015.08.001
Durán, E., Andújar, J. M., Segura, F., Barragán, A. J. (2011) A high-flexibility DC load for fuel cell and solar
arrays power sources based on DC–DC converters, Applied Energy, 88, 1690-1702. https://doi.org/10.1016/j.apenergy.2010.11.002
Erickson, R.W., Maksimovic, D. (2004) Fundamentals of power electronics. 2nd Edition, New York, Kluwer
Academic Publishers.
Haibing, H., Qian, Z., Xiang, F., Shen, Z. J. and Batarseh, I. (2011) A single stage mi-cro-inverter based on a three-port flyback with power decoupling capability. Energy Conversion Congress and Exposition (ECCE), IEEE, 1411-1416.
Honadia, P.A.A., Barro, F.I. and Sané, M. (2018) Performance Analysis of a Boost Converter with
Components Losses. Energy and Power Engineering, 10, 399-413. https://doi.org/10.4236/epe.2018.109025
Kesraoui, M., Korichi, N. and Belkadi, A. (2011) Maximum power point tracker of wind energy conversion system. Renew Energy; 36, 2655-2662. https://doi.org/10.1016/j.renene.2010.04.028
Li, Q. and Wolfs, P. (2008) A Review of the Single Phase Photovoltaic Module Integrated Converter Topologies With Three Different DC Link Configurations. IEEE Trans. on Power electron. 23, 320-1333.
Li, W., Lv, X., Deng, Y., Liu, J. and He, X. (2009) A Review of Non-Isolated High Step-Up DC/DC Converters in Renewable Energy Applications, 24th Annual Conference and Exposition of the IEEE, Applied Power Electronics Conference and Exposition, Washington DC, 15-19 February 2009,364-369.
Mahela, O. P., Shaik, A. G. (2017) Comprehensive overview of grid interfaced solar pho-tovoltaic systems, Renewable and Sustainable Energy Reviews, 68, 316-332. https://doi.org/10.1016/j.rser.2016.09.096
Petreuş, D., Daraban, S., Ciocan, I., Patarau, T., Morel, C. and Machmoum, M. (2013) Low cost single stage micro-inverter with MPPT for grid connected applications, Solar Energy, 92, 241-255. https://doi.org/10.1016/j.solener.2013.03.016
Rajesh, R., Mabel, M. C. (2015) A comprehensive review of photovoltaic systems, Re-newable and Sustainable Energy Reviews, 51, 231-248. https://doi.org/10.1016/j.rser.2015.06.006
Sadek, U., Sarjaš, A., Svečko, R., Chowdhury, A. (2015) FPGA-based control of a DC-DC boost converter, IFAC-PapersOnLine, 48, 22-27. https://doi.org/10.1016/j.ifacol.2015.08.102
Shuhui, L., Timothy, A. H. and Dawen, L. (2011) Fei H. Integrating photovoltaic and power converter characteristics for energy extraction study of solar PV systems. Renew Energy; 36, 3238-45. https://doi.org/10.1016/j.renene.2011.02.016
Taghvaee, M. H., Radzi, M. A. M., Moosavain, S. M., Hizam, H. and Marhaban, M. H. (2013) A current and future study on non-isolated DC–DC converters for photovoltaic applications, Renewable and Sustainable Energy Reviews, 17, 216–227. https://doi.org/10.1016/j.rser.2012.09.023
Ternifi, Z. E. T., Petit, P., Bachir, G. and Aillerie, M. (2017) New Topology of Photovoltaic Microinverter based on Boost converter. Energy Procedia, 119, 938- 944. https://doi.org/10.1016/j.egypro.2017.07.106
Variath, R. C., Andersen, M. A. E., Nielsen O. N. and A. Hyldgard. (2010) A review of module inverter topologies suitable for photovoltaic systems. Proc. IEEE IPEC, 310-316.
Wang, T. and Tang, Y. (2013) A High Step-up Voltage Gain DC/DC Converter for the Micro-Inverter. IEEE 8th Conference on Industrial Electronics and Applications (ICIEA), 1089-1094.
Winder, S. (2017) Power Supplies for LED Driving, 2nd Edition, Newnes.
Wu, G., Ruan, X. and Ye, Z. (2018) Non-isolated high step-up DC–DC converter adopting auxiliary capacitor and coupled inductor, J. Mod. Power Syst. Clean Energy, 6, 384–398. https://doi.org/10.1007/s40565-017-0342-8
Wu, K. (2016) Power Converters with Digital Filter Feedback Control, Academic Press. Wu, K. C. (1997) Pulse Width Modulated DC-DC Converters, Springer US, 1997. https://doi.org/10.1007/978-1-4615-6021-0